Comprehensive Analysis of Artificial Organs and Perspectives of their Development
- biologicalfervor
- Sep 3, 2024
- 26 min read
Written By: Veres Yuliia, Semkiv Anna, Vovk Alexandra
Introduction
The rapid advancement of technology has enabled significant progress in developing artificial organs, leading to their increasing integration into medical practice. It has been observed and explored crucial advancements in developing new materials and manufacturing methods that increased the general biocompatibility and endurance of manufactured organs, leading to the sprawling spread of obtaining artificial organs. In contradiction to the transplantation of human organs, the transplantation of artificial organs doesn’t require additional time for donor seeking and matching. Considering the advancements mentioned above, it has been decided to conduct a comprehensive literature analysis, investigating advancements in recently developed methods of manufacturing, comparison of some frequently used materials, analysis of the operating principles and clinical utility of a certain kind of artificial organs depending upon specific factors and demands, and assessment of potential challenges of application. Additionally, a sociological survey was conducted to estimate the general perception of using artificial organs in society.
General Information about Artificial Organs
Types of Artificial Organs
Depending on the way of manufacturing and used materials, artificial organs, in general, are classified into 3 large categories[4].
Mechanical artificial organs, respectively, do not contain any biomaterials and are fully composed of synthetic materials (particular kinds of modified plastic) and metals. The first transplanted artificial organs (heart and kidney), invented by Willem Kolf, were made of metal [1]. Thus, mechanical artificial organs initiated the beginning of an era of artificial organs by prompting scientists to explore new ways of manufacturing and exploiting artificial organs. Although some mechanical organs may not completely mimic inner organs in our body due to features of the materials used, they are highly used to replace external organs such as absent limbs (prostheses of legs, feet, elbows, hands, etc.) and to replace damaged or absent bones or joints (titanium plates for cranioplasty of the skull [2], artificial knee joint [3], etc.)
Biomechanical artificial organs, properties and characteristics of which are, consequently, a combination of particular properties of mechanical and biological ones because of the presence in their structures of both mechanical (metal or plastic parts) and living parts (cells, etc.)
Biological or bioartificial organs are built from artificially synthesized biodegradable biopolymers or living cells. In contradiction to mechanical organs, they are not as last because this type of organ, similarly to human organs, is more exposed to the influence of the body's inner processes. At the same time, they have a lower level of threat to cause an immunological reaction of rejection after transplanting to a human's body[5]. Not less important is the tendency to manufacture artificial using principles of physiology of corresponding organs to ensure the effect of mimicry. This approach makes it possible to create bioartificial organs that the organism cannot distinguish from the usual one, avoiding reactions of rejection or non-acceptance[5].
Artificial Sensory Organs
Artificial sensory organs are prosthetic devices designed to send visual or auditory information to the brain through electrical stimulation of the optic or auditory nerves, aiding visually impaired or hearing-impaired individuals.
Retinal implants or bionic eyes consist of an array of electrodes implanted in the eye that stimulate residual visual nerves with electrical signals, creating visual images. These implants translate images from a miniature camera into electrical impulses. Several commercially available systems, such as Alpha AMS, are currently in use, and also the Boston Retina Implant Project (BRIP) has developed a 15-channel implantable stimulator[7]. Moreover, it must be said that The Argus II was developed by Second Sight, a company based in Sylmar, California. It is an epiretinal implant that has been approved for sale in both the United States and Europe. The mechanism consists of a pair of glasses with a mounted camera connected to a small processing unit; its system uses a camera mounted on special glasses that sends a signal to an electronic receiver with 60 electrodes implanted inside the eye[8].
Cochlear implants replace damaged hair cells in the inner ear, converting sounds into electrical signals to stimulate the auditory nerve. Various commercial systems are available from manufacturers like Cochlear, MED-EL, Advanced Bionics, and others.[9]
Olfaction is addressed by the Bioelectronic Nose. It's an experimental technology where an array of sensors detects odors and transmits data to the brain via implanted electrodes, still in the laboratory research stage.[10]
Developments are also underway for prosthetic tactile limbs. Implanted neural stimulators may be capable of transmitting signals for touch, pressure, temperature, and other tactile sensations to the brain, partially restoring the sense of touch.
Artificial Hearts
An artificial heart is a technological device designed to support circulation. It completely replaces the removed biological heart, taking over the function of pumping blood through the circulatory system. It's intended for patients with end-stage heart failure as a "bridge" to heart transplantation or as a permanent implant.[11]
The concept of an artificial heart emerged in the 1960s, but realizing this goal proved extremely challenging. In the 1980s, American scientist Dr. Robert Jarvik and his team at the University of Utah developed one of the first fully implantable artificial hearts, the Jarvik-7.[12] Around the same time, Russian scientist Vladimir Demikhov performed the first successful implantation of an artificial heart in a human.[13]
The French company Carmat created a bioprosthetic heart using biological and synthetic materials in 2013. Trials showed months of functionality. Today, artificial hearts are still in clinical trial stages. The main challenges are ensuring long-term durability, and reducing the risk of thrombosis, and infections.[14]
Under the term "artificial heart," the following technical devices are understood:
- Ventricular assist devices (VADs) implanted in the body, intended to replace the heart muscle and improve the patient's quality of life.[15]
- Artificial heart valves are materials used to replace damaged heart valves, often seen in severe heart valve diseases. A mechanical heart valve is usually more durable than a bioprosthesis but carries a higher risk of thrombosis formation on the metal surface.[16]
- Devices for the maturation of heart tissue, enhancing the viability of implants, such as heart-specific extracellular matrices made from animal-derived bio-inks or organ-specific decellularized extracellular matrix (ECM).[17]
Artificial Kidneys
An artificial kidney is a medical device developed to replace the functions of kidneys in people with chronic kidney failure.[6]
It is a portable device for hemodialysis outside a medical facility. It is intended to purify blood from excess water, dissolved substances, and toxins in patients with kidney failure.
The first artificial kidneys were developed in the 1940s. One of the prototypes was created by the German scientist Willem Kolff during World War II. This device allowed for blood filtration and removal of excess substances, emulating kidney functions. Although imperfect, this development became the prototype for modern innovations in this field.[1, 18]
Currently, there are several types of artificial kidneys:
- Wearable artificial kidney (WAK) systems. «The WAK can be ergonomically adapted to the patient's body for maximum comfort while worn».[19]
- The device weighs less than 10 pounds, operates on 9-volt batteries, and uses less than 400 ml of fluid. Compare this to the standard dialysis machine that clocks in at over 300 pounds and uses 120 liters of water".
- Portable hemodialysis systems: This is the most common type of artificial kidney. They are designed for hemodialysis at home. They consist of a dialyzer, blood circuit, dialysis solution, and control system. They provide mobility but require connection to a water and electricity source.
- Dialysate regeneration systems: They clean and regenerate used dialysis solution for multiple uses, reducing fluid consumption.
- Implantable bioartificial kidneys: These are experimental devices implanted in the patient's body and function similarly to a real kidney. Scientists are currently only working on creating such a bioartificial kidney. It will consist of living nephrons (functional units of the kidney) placed in a biocompatible membrane. This device should function similarly to a real kidney.
Classification of Organ Manufacturing Technologies
Depending on the processing mechanism, bioartificial organ manufacturing technologies can be classified into groups[4]:
Two-nozzle 3D bioprinting is an advanced technique used in bioprinting, specifically for creating complex tissue structures. In bioprinting, bio-inks are used, which are materials containing living cells. These bio-inks can be different types of cells encapsulated within a supportive matrix. The Need for Multiple Bio-Inks is to mimic the complexity of real tissues, which often require multiple bio-inks. For example, for printing a blood vessel, we might need endothelial cells (lining the vessel) and smooth muscle cells (for the vessel wall).
Each bio-ink has specific properties and functions. Traditional bioprinters use more than one print head to switch between different bio-inks during the printing process [4].
In two-nozzle 3D bioprinting, there is a single printhead with two interchangeable nozzles.
These nozzles allow us to switch between different bio-inks seamlessly. The key is to minimize the transition distance when switching from one bio-ink to another.
With two nozzles, we can achieve continuous deposition of different bio-inks,
this ensures that the transition between bio-inks is as smooth as possible, maintaining accuracy and structural integrity.
Additive combined molding is an advanced technology that includes inside-out heterogeneous cell integration patterns in a layer-by-layer increasing manner. It is a typical semi-automated inside-out engineering technique regarding the predefined scale-up molds [4].
This method is very accurate, has high cell density, and has an easy eradication of immune response. As it is model-dependent, the creation of matrices is necessary.
Decellularized organ regeneration is an advanced technology, which is used to isolate the extracellular matrix (ECM) of a tissue while completely removing its inhabiting cells. The resulting ECM scaffold retains the natural architecture and composition of the original tissue. Let’s delve into the details. Decellularization aims to harvest the ECM for the regeneration of various tissues and organs. By using decellularized scaffolds, researchers hope to address the critical shortage of donor organs. Decellularized ECM preserves the natural microenvironment features that are essential for tissue function. It offers better biocompatibility compared to synthetic compounds. The ECM scaffold retains cell attachment sites, allowing seeded cells to adhere and grow [6].
Methods for Decellularization:
Chemical Decellularization: Involves using detergents, enzymes, or acids to remove cellular components while preserving the ECM.
Physical Methods: These include freeze-thaw cycles, mechanical agitation, and pressure changes.
Combination Approaches: Some protocols combine chemical and physical methods.
Aspects of Applying the Materials for Manufacturing Artificial Organs
The principles of functioning of artificial organs strictly correspond to the specific kind of artificial organs and materials of which the specific organ is made. In the chapter below, we have considered 3 general categories on which, in general, artificial organs are divined. By this classification, we can rely on considering the peculiarities of the functioning of each organ.
The functioning of mechanical artificial organs completely bounds to the characteristics of metals (such as iron, titanium, aluminum, stainless steel, and gold) and some smaller amounts of other enamine polymers (such as carbon-, nitrogen- and oxygen-containing compounds) [20] which were used for manufacturing and the applied technological schemes during the process of manufacturing [4]. By this, people avoid using only one certain type of metal, applying the precise correlation of metals’ content in their alloys to meet all the requirements for certain artificial organs.
In general traits, applying of artificial organs is strictly restricted by the biocompatibility of the materials from which they are made[4]. In contradiction to biomaterials, neither metals nor other enamine materials are biodegradable, so they cannot show higher biocompatibility than other precursors among biomaterials. Nonetheless, simultaneously with low indicators of compatibility of metals and other enamine compounds, these materials demonstrate the high strength of their fibers, the ability to endure harsh strain, and a noticeable resistance to physical deformations. Likewise, one of the properties that conditioned the spread applying of metals is their elastic deformation - the ability of material to instantaneously return to primer dimensions, size, and form, if the time of interaction with outer factors was relatively short. Metal alloys and some other enamine compounds also demonstrate high electrical conductivity indicators, enabling us to apply these materials to the inner mechanisms of artificial organs.
Interestingly, some metal alloys can possess properties that allow them to return to their primary form through the shape memory effect. Among the alloys, exactly the Nickel-titanium (Ni-Ti) alloys, sometimes called nitinol, most efficiently demonstrate this property of metals. This peculiarity allows the application of Ni-Ti for medical purposes, such as using these alloys for the fixation of broken bones or using Ni-Ti alloys in artificial kidney pumps.
In this manner, in medicine, we use mechanical artificial mostly in cases, when we prioritize physical properties, such as tensile strength, resistance to deformation, and abrasion resistance[20]. This leads us to the consequence that we can use artificial organs, made of metal alloys and other enamine compounds, for outer prosthetics of amputated limbs, where biocompatibility isn’t demanded, and physical properties are preferred, or we also can use these materials for creating the inner mechanism of biomechanical organs, where the outer part of an artificial organ which interacts straightforward with the human body and its metabolic process will be biocompatible unlikely to the inner mechanical component.
Biological tissues and cells, as a component of artificial organs, are highly used for manufacturing biological artificial organs. The organs that are manufactured from those materials are showing us incredibly high biocompatibility and biodegradability and low or almost absent immune reaction to organism rejection. Besides biological tissues and cells for manufacturing biological artificial organs, we might also use Biodegradable Scaffolds (such as collagen, chitosan, and gelatin), some synthetic biodegradable polymers (polylactic acid (PLA), polyglycolic acid (PGA), polycaprolactone (PCL), and polyethylene glycol (PEG))
Biomechanical artificial organs simultaneously combine itself peculiarities of bioartificial and mechanical organs due to the aspects of their structure. In this way, this hybrid kind of artificial organs can incorporate high indicators of physical properties, such as high tensile strength, resistance to fracture due to the influence of a high load of outer factors, etc. Also, it can reveal high measures of biocompatibility and biodegradability because of the particulate contents of biological materials. According to current observations, exactly this category of artificially made organs is the most applied in regenerative medicine owing to the following advantages, such as enhanced Functionality, Improved compatibility, durability and longevity, self-repair and adaptation, and reduced Immunosuppression.
Challenges of Using Artificial Organs
Immunological Reaction
It is mainly thought that bioengineered organs might escape immunological reactions because of the specific materials they are made of. However, potential immune responses have not been studied in detail.
Antigens that are implanted in the body invariably cause a local immunological reaction. The biological reaction of these organs is determined by several factors, including surface topography, chem composition, speed of resorption, type of dissolution product, and mechanical properties [20].
The fundamental principle of immunochemical techniques is that a specific antibody will bind to a specific antigen. Antibodies are Y-shaped molecules consisting of four polypeptide chains, each consisting of two identical light chains and heavy chains, linked together by disulfide bridges [20].
The immune responses to bioengineered organs can be classified based on the component of the organ that is being recognized by the immune system.
Here are the main types:
Cell-specific immune responses: involve the host’s immune response toward different progenitor cells used in the development of bioengineered organs [21].
Immune responses to non-self biological scaffolds: they are recognized as foreign substances by the immune system. As early as nanoseconds after implantation, the tissues, proteins from blood, and intestinal fluids are adsorbed to the surface of the biomaterial. This causes the activation of the complement system, coagulation cascade, platelets, immune cells, and triggers an inflammatory response [22].
Protein-specific immune responses: involve modifying the amount or function of extracellular proteins in the immune system. This could be a response to the proteins present in the bioengineered organ or those produced as a result of the interaction between the organ and the host’s immune system.
These immune responses could have significant implications for the successful integration and function of bioengineered organs in recipients. In most cases, the immune response can be managed via immunosuppressant therapy, but it is still a major problem.
Terms of Explanation
The use of artificial organs requires consideration of corrosion effects to ensure durability and functionality. Corrosion is one of the most common issues with metals, occurring when oxidation, a process in which metal combines with oxygen, takes place. Corrosion adversely affects and deteriorates the condition of metals. To determine methods to prevent corrosion, it is necessary to understand how different types of corrosion occur.
Galvanic Corrosion
Galvanic corrosion is a type of corrosion that occurs when two dissimilar metals are electrically connected in a corrosive environment. This principle is similar to how dry cell batteries work: two metals in contact with a liquid electrolyte, such as body fluid. The corrosion rate of one metal increases while the other decreases compared to when they are not in electrical contact. The metal that corrodes faster becomes the anode, and the other the cathode, creating an electrical potential between them. To prevent galvanic corrosion, metals can be electrically insulated from each other, or combinations of metals that do not significantly affect corrosion rates can be selected [23].
Crevice Corrosion
Crevice corrosion is a highly localized form of corrosion in crevices and shielded areas, such as joints or under gaskets. The rest of the surface usually remains undamaged. Stainless steel is particularly susceptible to this type of corrosion. Other materials, such as titanium, are resistant to this type of corrosion in specific environments. The crevice is usually hundredths of a millimeter in size, allowing liquid entry but maintaining a stagnant zone. Initially, corrosion occurs uniformly over the entire surface, but over time, oxygen in the crevice depletes while the metal continues to dissolve. This leads to an accumulation of positive charge balanced by chloride ions migrating into the crevice. Metal salts hydrolyze in water, forming an insoluble metal hydroxide and a free acid, accelerating the corrosion process. To prevent crevice corrosion, avoiding crevices and adding 2% molybdenum to 18-8 stainless steel to prevent pitting, which is similar to crevice corrosion, is effective [24, 20].
Stress Corrosion Cracking
Stress corrosion cracking results from the combined action of tensile stress and a corrosive environment. Both conditions are necessary for its occurrence; some materials may hardly corrode in a corrosive environment unless stress is present. Small cracks form and propagate perpendicular to the stress, eventually leading to failure. Most alloys are susceptible to this type of corrosion in specific environments, for example, stainless steels in acid chloride solutions and titanium alloys in seawater. Fortunately, the number of environments in which a specific alloy will crack is small. The best way to reduce or prevent this type of corrosion is to reduce the stress on the metal. Alternatively, the metal can be separated from the corrosive environment by a coating, or a different alloy can be used [25].
As an example, the NEXT project aims to develop artificial pancreatic islets that avoid the recipient's immune response through vascular chimerism and physical immune shielding. Two exploitation strategies are envisaged:
- Long-term exploitation strategy
for the NEXT artificial pancreatic islet expected to reach the market 5-7 years after project completion.
- Short-term exploitation strategy
for intermediate results achieved during the first 36 months, expected to reach the market within 1-2 years.
Long-term Exploitation Strategy
The ultimate objective of the NEXT project is to develop a chimeric artificial pancreatic islet capable of escaping the recipient's immune response through vascular chimerism and physical immune shielding. This technology falls under the advanced therapy medicinal product (ATMP) category according to Directive 2001/83/EC as amended [26, 27].
Products of Secretion
Secretion, in biology, production and release of a useful substance by a gland or cell. Products of secretions include the gastrointestinal tract which secretes digestive enzymes and gastric acid, the lungs which secrete surfactants, and sebaceous glands which secrete sebum to lubricate the skin and hair.
Secretions of Natural Organs:
- Digestive System: The digestive tract secretes mucus, which protects the stomach lining from acidic digestive juices and facilitates the passage of feces.
- Respiratory System: The respiratory tract produces mucus to trap inhaled bacteria and particles, which are expelled through coughing or swallowing.
- Endocrine System: Endocrine glands secrete hormones that regulate diverse bodily functions, such as growth, metabolism, and reproduction.
Secretion of Artificial Organs:
Encapsulating secretory cells within microbeads or capsules could allow for the controlled release of specific substances. Also, engineering biomimetic materials that mimic the natural structures and functions of tissues could enable artificial organs to secrete substances in a physiologically relevant manner.
- An artificial pancreas could secrete insulin to regulate blood sugar levels, potentially addressing diabetes.
- An artificial liver could secrete bile to aid digestion and bilirubin to eliminate toxins, potentially treating liver failure.
- An artificial kidney could filter blood and remove waste products, providing a viable alternative for patients with kidney disease. [28]
Innovations in the Field of Creation of Artificial Organs
Kidney Innovations
Patients with kidney diseases today face a grim reality: within three years of starting dialysis, most of them will die. To address the urgent need for improved outcomes, KidneyX has leveraged open innovation. Last fall, KidneyX launched the Artificial Kidney Prize, a competition aimed at accelerating the development of artificial kidneys for human clinical trials. Phase 1, designed and managed by Luminary Labs, invited innovators to submit solutions that enhance the functionality, efficiency, and/or reliability of artificial kidneys. [29]
Subsequently, HHS and ASN announced six Phase 1 winners, each receiving $650,000:
- David Cooper, University of Alabama: Genetically engineered pig kidneys designed to reduce the likelihood of rejection, providing more viable donor kidneys.
- Imec USA Center for Nanoelectronics Design: A miniature, wireless system for toxin removal, suitable for implanted, wearable, portable, or bedside artificial kidneys.
- Makana Therapeutics: Genetically modified pig kidneys to increase the supply of transplantable organs by overcoming antibody barriers to xenotransplantation.
- University of California, San Francisco: An implanted bioartificial kidney enabling continuous blood processing and waste directed to the bladder, allowing freedom of movement.
- University of Washington Dialysis Innovation Center: A wearable continuous hemodialysis device providing greater mobility.
- American Kidney Research Corporation: A wearable artificial kidney that requires no water for filtration, significantly reducing device weight.[30]
Heart Innovations
A new kind of artificial heart that combines synthetic and biological materials, sensors, and software to detect a patient’s level of exertion and adjust output accordingly, is set to be tested in patients at cardiac surgery centers in Europe and the Middle East. If the bioprosthetic device made by Paris-based Carmat [31] proves safe and effective, it could be used for patients waiting for heart transplants [32]
China announced a new artificial heart built on rocket technology, currently undergoing testing. The "aerospace heart" uses magnetic and liquid levitation from a rocket system and is expected to enter clinical trials in the coming years. [33]
In Switzerland, researchers have developed a soft heart made of silicone using innovative 3D printing methods. It has left and right ventricles, pumps blood-like fluid, and weighs about the same as a natural human heart. [34]
Queensland researcher Daniel Timms’ titanium mini-heart, small enough to fit into a child's chest but powerful enough to support an adult, has become a leader in artificial heart technology. Liver Innovations [35]
Liver Innovations
Researchers at Texas A&M University have created an artificial liver using lab-grown human liver cells to perform detoxification and protein synthesis functions. This development could become an alternative to liver transplantation for patients with liver failure [36]
Lung Innovations
Scientists at the University of Pittsburgh have developed artificial lungs that can operate for extended periods without external power sources, a potential lifesaver for people needing lung transplants.[37]
Pancreas Innovations
Medtronic has received FDA approval for its artificial pancreas, an implantable device that can automatically regulate insulin levels in patients with type 1 diabetes.[38]
Sensory Organ Innovations
In sensory organs, advances have been made in developing artificial retinas and cochlear implants. These devices use electrical stimulation to transmit sensory information to the brain, enabling the restoration of vision and hearing in patients. For example, artificial retinas can help patients detect motion, locate objects, and follow pathways. [39, 6]
3D Bioprinting
3D bioprinting technology is actively used to create various biological structures, including liver tissues, hearts, and vascular networks. This technology allows for the creation of complex anatomical structures that can be used for transplantation and drug testing. Recent studies have shown that the use of bio-printed tissues can significantly improve transplant outcomes and reduce the risk of rejection. [40]
Artificial Nerves and Brain-Computer Interfaces
Artificial nerves and brain-computer interfaces (BCIs) are also under development to compensate for spinal cord injuries and control paralyzed muscles. These technologies use signals synthesized from patients' brain and muscle activity to restore functions lost due to injury or disease. [6]
OpenWorm Project
The OpenWorm project aims to create a complete digital model of the C. elegans nematode, including its nervous system. This ambitious task could help us better understand the human brain and develop new treatments for neurological diseases. [41]
Ethical Problems
Biomedical technologies are rapidly evolving, which may cause new challenges in the ethical area. That’s why there are 3 general moral principles:
The principle of respect for autonomy: the decision about using bioengineered organs must be made by the patient, without violence of his rights. [20, 43]
The principle of beneficence: doing good or acting in the best interest of the patient. [20, 44]
The moral principle of justice: similar cases should be treated alike. However, there is a concern as everyone has a unique genome and physiological processes, different financial situations, and moral beliefs. [20]
Along with advancements in biomedical engineering technologies, there arise moral uncertainties. There are main reasons that cause ethical problems in using artificial organs:
The type of biomanufactured organ (mechanical, biomechanical, bioartificial) and specific materials it is made from (different metals, biopolymers, tissues): any kind of artificial organ will be an antigen to the recipient's body. That means that each of them has its pros and cons in certain criteria, but the main issue is the moral preferences of the recipient.
Patient safety and risk assessment: every manipulation with biomedical devices has some risks, which must be evaluated to ensure the safety of patients. [42]
Informed consent and human testing: informing patients and participants about all aspects of taking part in clinical trials and getting their consent. [42]
Cloning tissues and stem cell research: it might be evolutionary harmful. The potential loss of genetic material and the mutations it can bring to later generations haven’t been studied yet. It may be difficult to obtain the consent of donors for taking material and conducting clinical research. [46, 47]
Human enhancement: genetic manipulation and the development of prosthetic organs, raises ethical questions about the definition of being human. [45]
Accessibility and affordability: ensuring that medical technologies are accessible and affordable to all who need them.[45]
Animal testing: prevention or at least reducing suffering in other species. [46]
Environmental impact: promoting sustainability, minimal impact of bioengineering processes and products. [45]
Problems that are not related to biomedical engineering, but may arise due to the use of computer technologies or other factors: patient privacy and data security, intellectual property rights and the exchange of discoveries between institutions, and equal distribution of scarce medical resources.
We surveyed to find out how society feels about the ethical problems of biomedical engineering. We surveyed people from different countries, age groups, occupations, and levels of awareness of the research topic.
All the responders highly rate the importance of the type of materials for creating artificial organs. 65% indicate the privilege of using artificially grown(cultivated) cells, while 35% believe that biopolymers are a good source. No one rated metals as the best material.

Figure 1. Preference for some materials used in artificial organ manufacturing
Respondents were asked to indicate their trust level in using artificial organs, their answers ranged from 1 to 10. (1 – don’t trust, 10 – completely trust). Based on the diagram we can see a big range in trust levels to these medical developments.

Figure 2. Trust level in the use of artificial organs
Most of the surveyed people (75%) would agree to an artificial organ implantation
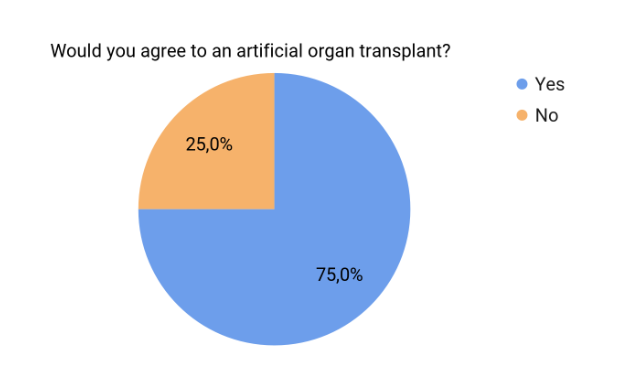
Figure 3. Rate of agreeableness to artificial organ transplants
Many of the study participants indicated possible risks of using artificial organs for patients:
The greatest risk is the reaction of the immune system and the production of antibodies, and the issue of blood clotting during passage through artificial organs also increases.
Even though rarely it may be hazardous to human health, its positiveness is more than 70% because of which many professional people check them.
I think the risk of using artificial organs from cultivated cells can be pretty low because the cells can be manipulated in such a way that the mutations can occur until the full organ is formed and transplanted.
It has risks, but if it helps improve lifestyle then I do not see any problem.
It might lead to a paralysis of some body parts.
Responders were asked whether the following situation is acceptable, based on their point of view: when the latest medical developments do not have time to go through all of the clinical research they can be used with the written consent of the patient.
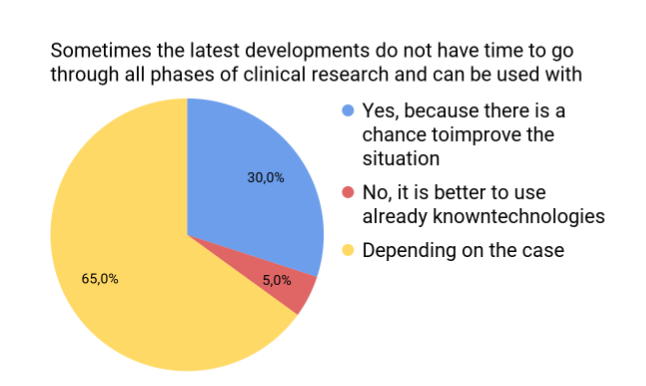
Figure 4. Responses for modeling the following situation
There are assumptions on how these technologies can change humanity:
Help save lives
Instead of natural abilities, some individuals would have heightened advantages
It is a set of technologies used to change the genetic makeup of cells, including the transfer of genes within and across species boundaries to produce improved or novel organisms.
Can make their life better, though it could have unintended consequences.
It can evolve humans and rapidly advance medical sciences and tech
There are ideas for overcoming certain ethical issues regarding artificial organs
Spreading awareness and making access to these resources equal for everyone
State programs with financial assistance
Volunteer funds
International organizations
Education, first of all. Tolerating such things and spreading quality, true information, to convince people that this is to some extent salvation. Because people are used to organ transplants the ethical issue becomes less acute. so later, everything will be the same.
According to survey findings, the material type of artificial organs plays an important role, and each has certain risks. The trust level in using artificial organs varies from 1 to 10, and most responses are from 4 to 8. The bigger part of survey participants indicate their agreeableness to an artificial organ transplant. There are many responses about the possible risks of using such medical developments when they did and didn’t pass all stages of clinical research. However, some are inclined to believe that in the future these technologies can lead to improvement of human life and further evolution of science.
To overcome ethical problems in the field of biomedical engineering, it is necessary to inform society widely about available medical technologies, making access to them equal to everyone, launching international as well as state programs with financial assistance, and collecting volunteer funds.
Conclusion
This research paper summarizes the primary information about modern technologies for artificial organ manufacturing and the challenges of implementing their usage in society from comprehensive literature and sociological survey analysis.
Artificial organs, overall, are classified into 3 categories: mechanical, biomechanical, and biological bioartificial organs. Such classification is fully based on the manufacturing ways and applied materials. The choice of a certain kind of artificial organ depends on the range of preferred qualities and functions, expected to be executed: mechanical artificial organs demonstrate substantial resistance to physical factors, bioartificial organs present high indicators of biocompatibility and are highly biodegradable, and biomechanical ones are partially combining some properties of kinds, listed before.
Along with the rapid evolution of artificial organs there appeared many technologies for their manufacturing, which were classified into 3 groups: two-nozzle 3D bioprinting, additive combined molding, and decellularized organ regeneration. This division is done depending on laboratory equipment, types of matrices, and methods of working with cells.
The functioning of artificial organs is defined by manufacturing technologies and applied materials, influenced by the characteristics of used materials. The mechanical artificial organs, made out of alloys, offer advanced potential for use because of enhanced tensile strength, resistance to fracture, measure of biocompatibility, and biodegradability. To avoid the abrupt immune response after transplanting bioartificial organs inside the human body, it's highly recommended to use bioartificial organs to decrease the expected abrupt immune response organs, made of biological tissues and tissues, biological scaffolds, and some synthetic biodegradable polymers.
Due to the complexity of the human body and the variety of physiological processes, the usage of artificial organs may be limited. The main challenge of organ transplantation is the immunological reaction, which is individual, but still can be estimated and divided into groups depending on the immune response to materials of artificial organs made from.
The lifespan of artificial organs is highly dependent on their specific type and the materials used. While many last up to 10 years, ongoing research aims to extend this duration. Various factors can shorten the lifespan of implants, including wear and tear, infections, blood clot formation, and rejection by the body. However, one of the primary challenges in the long-term use of artificial organs is material degradation due to corrosion. This can manifest in several forms, such as crevice corrosion, galvanic corrosion, and stress corrosion cracking.
The secretions produced by artificial organs play a central role in their function and interaction with the body. They can affect the organ's functions, its interaction with other body systems, and the overall health of the patient. Therefore, to further improve artificial organs, it is necessary to study the processes of secretion and their regulation in detail, as well as to assess the potential risks.
Innovations in biomedical engineering are taking a huge step away from today's donor dependence and revolutionizing medicine by offering new methods of treatment and restoring human body functions. Advances such as tissue bioprinting, stem cell utilization, and improved biomaterials bring us closer to creating more effective and durable artificial organs. These advancements open up possibilities for improving the quality of life for patients suffering from severe diseases so the development of artificial organs does not stand still.
The implementation of recent advancements in biomedical engineering in our lives raises a series of ethical dilemmas. Before turning to the center of the problem there were described the main moral principles of Biomedical Engineering. Then there was a description of the main reasons that cause problems. Based on this information an international survey was conducted to estimate the opinions of individuals on these problems. Participants were asked multiple questions regarding the materials artificial organs are made from, safety-risk ratio, trust level, agreeableness to an artificial organ transplant, impact of medical technologies on global society, and ways to solve those ethical problems. Our main findings are presented in the figures above. Mainly, there is a lack of information regarding research topics, which leads to low awareness and misunderstanding of ethical concepts. So there is a huge need in carrying out public information work, developing organ manufacturing technologies, and influencing local authorities to make certain financial programs to help people in need to have equal access to medical advancements.
Bibliography
1. Cooley, D. A. (2009). In Memoriam: Willem Johan Kolff 1911–2009. PubMed Central (PMC). https://www.ncbi.nlm.nih.gov/pmc/articles/PMC2676591/
2. Aydin S, Kucukyuruk B, Abuzayed B, Aydin S, Sanus GZ. Cranioplasty: Review of materials and techniques. J Neurosci Rural Pract. 2011 Jul;2(2):162-7. doi: 10.4103/0976-3147.83584. PMID: 21897681; PMCID: PMC3159354.
3. Mohammad Azar Bargir, Nitin G. Phafat, Vijya Sonkamble,
A review of artificial knee joint by additive manufacturing technology to study biomechanical characteristics, Advances in Oral and Maxillofacial Surgery,
Volume 12, 2023, 100447, ISSN 2667-1476, https://doi.org/10.1016/j.adoms.2023.100447.
4. Wang X. Bioartificial Organ Manufacturing Technologies. Cell Transplant. 2019 Jan;28(1):5-17. doi: 10.1177/0963689718809918. Epub 2018 Nov 26. PMID: 30477315; PMCID: PMC6322143.
5. K Praveena, Manjunatha, Ankita Awasthi, Amit Dutt, Irfan Khan, Preeti Maan and Raghad Ahmed Hussien: Biomaterials for Artificial Organs and Organoids- A Comprehensive review. Issue E3S Web Conf; Volume 505, 2024 3rd International Conference on Applied Research and Engineering (ICARAE2023)Article Number01004 https://doi.org/10.1051/e3sconf/202450501004; Published online25 March 2024
6. MacNeil, D. (2023, December 12). Artificial Organs: Innovating to Replace Donors and Dialysis. The Scientist Magazine®. https://www.the-scientist.com/artificial-organs-innovating-to-replace-donors-and-dialysis-70907
7. Shepherd, R., Fallon, J., & McDermott, H. (2014). Medical Bionics. In Elsevier eBooks (pp. 327–341). https://doi.org/10.1016/b978-0-444-53632-7.01020-0
8. USC Eye Institute ophthalmologists implant first FDA-approved Argus II retinal prosthesis in western United States. (2014, August 27). Reuters. https://web.archive.org/web/20150105222606/http://www.reuters.com/article/2014/08/27/usc-eye-institute-fda-idUSnPn6JGDrT+9d+PRN20140827
9. Tzvi-Minker, E., & Keck, A. (2023). How Can We Compare Cochlear Implant Systems across Manufacturers? A Scoping Review of Recent Literature. Audiology Research, 13(5), 753–766. https://doi.org/10.3390/audiolres13050067
10. Dung, T., Oh, Y., Choi, S. J., Kim, I. D., Oh, M. K., & Kim, M. (2018). Applications and Advances in Bioelectronic Noses for Odour Sensing. Sensors, 18(2), 103. https://doi.org/10.3390/s18010103
11. Artificial heart. (2024, July 12). Wikipedia. https://en.wikipedia.org/wiki/Artificial_heart
12. Robert Jarvik, MD on the Jarvik-7 | Jarvik Heart Inc. (2021, February 6). Jarvik Heart Inc. |. https://www.jarvikheart.com/history/robert-jarvik-on-the-jarvik-7/
13. Matskeplishvili, S. (2017). Vladimir Petrovich Demikhov (1916–1998). European Heart Journal, 38(46), 3406–3410. https://doi.org/10.1093/eurheartj/ehx697
14. Stephane Piat. (2023). CARMAT 2022 Registration Document including Financial Report. Retrieved July 19, 2024, from https://www.victrex.com/-/media/downloads/literature/enterprise/changemaker_carmat_artificial-heart_literature_2023.pdf
15. Ventricular assist device (VAD) - Mayo Clinic. (2023, May 3). https://www.mayoclinic.org/tests-procedures/ventricular-assist-device/about/pac-20384529
16. 3 common types of artificial heart valves and pros and cons. (n.d.). Vinmec. https://www.vinmec.com/en/cardiology/health-news/3-common-types-of-artificial-heart-valves-and-pros-and-cons/
17. Kim, Byoung; Das, Sanskrita; Jang, Jinah 2020/07/31 Decellularized Extracellular Matrix-based Bioinks for Engineering Tissue- And Organ-specific Microenvironments 10.1021/acs.chemrev.9b00808
18. Malloryk. (2020, April 5). Medical Innovations: Under Occupation, the Development of Dialysis. The National WWII Museum | New Orleans. https://www.nationalww2museum.org/war/articles/medical-innovations-dialysis
19. Wearable Artificial Kidney (W.A.K.) | Dr. Victor Gura. (2024, June 6). Victor Gura, MD. https://drgura.com/wearable-artificial-kidney/
20. Hench, Larry L., et al. Biomaterials, Artificial Organs and Tissue Engineering. CRC Press ; Woodhead, 2005, http://www.crcnetbase.com/isbn/9780203024065.
21. Tripathi, A. S., Zaki, M. E. A., Al-Hussain, S. A., Dubey, B. K., Singh, P., Rind, L., & Yadav, R. K. (2023). Material matters: exploring the interplay between natural biomaterials and host immune system. Frontiers in Immunology, 14. https://doi.org/10.3389/fimmu.2023.1269960
22. Priyom Bose Immune Responses to Implants. (2021, December 17). AZoLifeSciences. https://www.azolifesciences.com/article/Immune-Responses-to-Implants.aspx
23. Galvanic Corrosion - AMPP. (n.d.-b). Higher Logic, LLC. https://www.ampp.org/technical-research/impact/corrosion-basics/group-1/galvanic-corrosion
24. Noël, J., Ebrahimi, N., & Shoesmith, D. (2018). Corrosion of Titanium and Titanium Alloys. In Elsevier eBooks (pp. 192–200). https://doi.org/10.1016/b978-0-12-409547-2.13834-x
25. Stress Corrosion Cracking - AMPP. (n.d.). Higher Logic, LLC. https://www.ampp.org/technical-research/impact/corrosion-basics/group-3/stress-corrosion-cracking
26. Cordis, C. (2018, April 9). New biomaterials enable the engineering of pancreatic islets from single cells. CORDIS | European Commission. https://cordis.europa.eu/article/id/219649-new-biomaterials-enable-the-engineering-of-pancreatic-islets-from-single-cells
27. Wang, Q., Huang, Y. X., Liu, L., Zhao, X. H., Sun, Y., Mao, X., & Li, S. W. (2024). Pancreatic islet transplantation: current advances and challenges. Frontiers in Immunology, 15. https://doi.org/10.3389/fimmu.2024.1391504
28. Zalevsky, Z., & Abdulhalim, I. (2014). Fabrication Aspects of Integrated Devices. In Elsevier eBooks (pp. 103–115). https://doi.org/10.1016/b978-0-323-22862-6.00004-9
29. Open innovation | Luminary Labs. (2024, April 17). Luminary Labs. https://www.luminary-labs.com/work/open-innovation/
30. Hibbard, J. (2023, June 19). 6 innovators advancing artificial organs | Luminary Labs. Luminary Labs. https://www.luminary-labs.com/6-innovators-advancing-artificial-organs/
31. Home - Carmat. (2024, July 15). Carmat. https://www.carmatsa.com/
32. Rojahn, S. Y. (2020, April 2). The Latest Artificial Heart: Part Cow, Part Machine. MIT Technology Review. https://www.technologyreview.com/2013/05/30/178282/the-latest-artificial-heart-part-cow-part-machine/
33. Pti. (2018, March 15). China develops artificial heart with rocket technology: Report. The Times of India. https://timesofindia.indiatimes.com/home/science/china-develops-artificial-heart-with-rocket-technology-report/articleshow/63312264.cms
34. This 3-D-printed artificial heart actually beats. (n.d.). СNN. From https://edition.cnn.com/2017/07/20/health/3-d-printed-heart-silicone-study/index.html
35. Riches, C. (2024, April 18). The world’s most advanced long-term artificial heart is on the cusp of implantation - create digital. Create Digital. https://createdigital.org.au/long-term-artificial-heart-implantation/amp/
36. Schattenberg, P. (2024, May 2). AgriLife Research-led study examines nonalcoholic fatty liver disease. AgriLife Today. https://agrilifetoday.tamu.edu/2022/10/04/agrilife-research-led-study-examines-nonalcoholic-fatty-liver-disease/
37. Wearable Artificial Lung to Be Developed at Pitt Through $3.4 Million Grant. (n.d.). Copyright 2010 University of Pittsburgh. https://www.news.pitt.edu/artificial_lung_grant
38. Medtronic’s long-awaited ‘artificial pancreas’ makes U.S. debut. (n.d.). FIERCE Biotech. From https://www.fiercebiotech.com/medtech/medtronic-s-long-awaited-artificial-pancreas-makes-u-s-debut
39. One Step Closer to Artificial Organs: Living Fossil Provides New Insight. (2023, January 22). SciTechDaily. https://scitechdaily.com/one-step-closer-to-artificial-organs-living-fossil-provides-new-insight/
40. Huang, G., Zhao, Y., Chen, D., Wei, L., Hu, Z., Li, J., Zhou, X., Yang, B., & Chen, Z. (2024). Applications, advancements, and challenges of 3D bioprinting in organ transplantation. Biomaterials Science. https://doi.org/10.1039/d3bm01934a
41. OpenWorm. (2024, July 26). Wikipedia. https://en.wikipedia.org/wiki/OpenWorm
42. Bioengineering Ethics: The Ethics of the Linkage Between Engineering and Biology. (2003). The Experiences and Challenges of Science and Ethics - NCBI Bookshelf. https://www.ncbi.nlm.nih.gov/books/NBK208734/
43. Łuków, P., & Różyńska, J. (2015). Respect for Autonomy. In Springer eBooks (pp. 1–12). https://doi.org/10.1007/978-3-319-05544-2_380-1
44. Margaret, L. (2013). Ethical and Legal Issues in Neurology. In Handbook of Clinical Neurology. Beneficence https://www.sciencedirect.com/topics/medicine-and-dentistry/beneficence
45. Challenges and Ethical Considerations in Biomedical Engineering. (2001, March 14). CWRU Online Engineering. https://online-engineering.case.edu/blog/challenges-and-ethical-considerations-in-biomedical-engineering
46. Daniel A. Vallero. Biomedical Ethics for Engineers. (n.d.). Google Books. https://books.google.com.ua/books?hl=uk&lr=&id=AeT56Pi8LFYC&oi=fnd&pg=PP1&dq=biomedical+engineering+ethics&ots=1bDIJUxhog&sig=LVmj_q7-2qWlZoHsbNpc_lkJ-3E&redir_esc=y#v=onepage&q=biomedical%20engineering%20ethics&f=false
47. Hyun, I. (2010). The bioethics of stem cell research and therapy. the Journal of Clinical Investigation/ the Journal of Clinical Investigation, 120(1), 71–75. https://doi.org/10.1172/jci40435
Comments